|
|
|
Research
Below you find brief summaries of our three research directions.
For research highlights please browse down our home page.
|
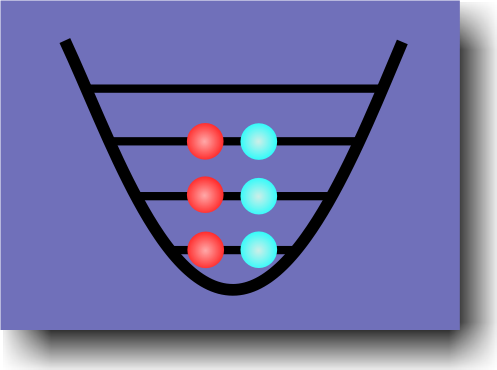 Fermi Mixture
The Fermi Mixture project is dedicated to study quantum many body-physics in fermionic and bosonic mixtures of ultracold quantum gases. While the main focus is on the two-species Fermi-Fermi mixture of lithium and potassium our experimental platform also offers to study Bose-Fermi mixtures that include rubidium. Having multi-wavelength optical trapping potentials and ultrastable magnetic fields for Feshbach resonances at hand the system is an ideal toolbox to simulate and explore many-body quantum phases that are not available to this extend of purity and control in other physical disciplines. Like spin mixtures of single-species fermionic gases the two-species Fermi-Fermi mixture proved to show enhanced lifetime in the strongly interacting regime due to the Pauli Exclusion Principle. This is the basis to study phenomena where the mass difference occurs as an additional parameter, like for example the BEC-BCS crossover with unmatched Fermi energies, or properties of heteronuclear dipolar molecules. We have produced a comparatively large sample of bosonic Feshbach molecules of lithium and potassium is with long lifetime. Precise measurements of the Feshbach resonance for the elastic collisional cross section, reveal the appareance of molecules even on the atomic side of the narrow resonance stabilized by the presence of fermionic atoms in the smaple. This can be seen as a first observation of a many body effect at a narrow Feshbach resonacne. The production of heteronuclear molecules is a milestone on the way to a Mott-Insulator of heteronuclear molecules in their rhovibrational ground state. Therefore, this system is a promising candidate to realize a quantum gas with long range non-isotropic dipolar interaction. The lithium-potassium combination seems quite favourable due to its comparatively large dipole momenet. We have installed electrodes inside our UHV vaccum chamber that will allow us to create an electric dipole moment of approximately 2 Debye, which corresponds to an interaction energy of 330 nK between dipoles on a typical optical lattice. Such energy would dominate the temperature scale of the sample, and hance pave the way to explore a wealth of quantum phenomena in optical lattices.
|
Fermions in Optical Lattices
The research objective of this project is to study quantum many-body physics using cold fermionic atoms in optical lattices. The focuses are on various two dimensional (2D) lattice configurations, motivated by the fact that many highly interesting but not well-understood phenomena in condensed-matter physics arise from 2D geometries. One example can be the realization of a graphene type of hexagonal optical lattice. Interactions can be tuned from non-interacting to strongly coupling through atomic Feshbach resonances. Together with other possibilities to tune the system, many-body states such as superfluid states, anti-ferromagnetic states, and so on can be experimentally accessed. In particular studying effects of disorder potentials in the presence of strong interactions can be studied well for the case of lithium. While setting up the experiment we have been studying as an intermediate milestone narrowband cooling of fermionic lithium-6 atoms. Due to its non-resolved hyperfine structure conventional sub-Doppler cooling techniques do not yield low temperatures. This hampers of the production of ultracold samples of lithium in the quantum degenerate regime, as with large temperatures only low densities are achieved and loading of small volume optical dipole traps is not efficient. We have been studying narrowband cooling by loading atoms from a standard magneto-optical trap (MOT) employing the D2 line at 671nm over into a MOT running at 323nm with a laser linewidth of approximately 40 kHz. This near UV light drives the 2S->3P transition for which the narrow linewidht gives rise to a Doppler temperature that is almost as low as the recoil temperature below 20 microkelvin. We have characterized various parameters of the trap and report high phase space densities at temperatures around 40 microkelvin. These results will facilitate efficient production of cold samples in an optical lattice in the future.
|
Laser Spectroscopy and Shared Frequency Comb
Motivated by our goals to produce ground state dipolar molecules by a coherent stimulated Raman adiabatic passage (STIRAP) we have been setting up two narrowband lasers which frequency difference is controlled by comparison to a frequency comb. Based on a comparatively simple optical resonator the linewidth of our lasers is brought into the sub-kHz regime sufficient for tuypical applications in molecular spectroscopy and STIRAP. For molecular spectroscopy we have investigated in an industry collaboration an interferometric stabilzation of the laser frequency that achieved MHz precision over a range of several tens of nanometers. For the purpose of STIRAP we employ a commercial frequency comb system based on a 1550 nm pulsed fiber laser. The frequency comb is stabilized on short timescales to one of our STIRAP lasers, while on long time scales it is tight to a reference signal obtained from a GPS receiver. Our frequency comb system is set up as a shared facility that can serve as a frequency reference for several other laboratories at CQT. The system comprises several sets of amplification, second harmonic generation, and photonic-crystal fibers. In this way several comb light sources are being produced in different spectral regions. This enables a variety of application lasers and experiments to benefit from this facility. For applications lasers with a wavelength outside the spectral range of the comb light we recently demonstrated a frequency comb assisted cavity transfer frequency stabilization scheme.
|
|
|
|